Table of Contents
Quantum computing is rapidly evolving as a transformative technology, with substantial advances in the commercial and academic sectors. According to Strangeworks, with a global quantum investment exceeding $30 billion in 2022 and the creation of 37 master’s programs in quantum technologies, its influence is growing in the public markets. Today’s practical uses of quantum computing include machine learning, cryptography, search algorithms, logistics, optimization, medicinal materials manufacturing, and others.
This rising prominence reflects a fundamental shift in our approach to challenging scenarios. The strength and speed of quantum computational technologies provide an unparalleled opportunity to address some of the toughest challenges in the industry. Today, our focus is on the pivotal role of quantum computing in the field of chemistry.
Computational chemistry searches to predict the physical properties and behaviors of molecules, and materials with quantum mechanics. Despite the costs that classical approaches have historically faced in precisely modeling complex systems, tremendous progress has been made via more sophisticated approximation methods. Consequently, computational chemistry has become integral to experiment analysis in chemistry, the pharmaceutical drug development process, and material optimization.
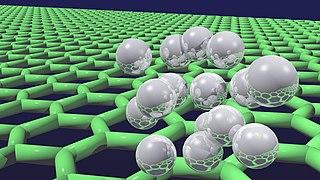
Quantum simulation, a vital tool in computational chemistry, is exemplified in this demonstration, which visualizes hydrogen atom delocalization over a graphene surface. © Wikimedia commons.
Two of the most critical areas in computational chemistry are the computation of electronic and vibrational structures of chemical systems. Given these problems’ inherent quantum mechanical nature, several quantum computational methods have been developed and are now actively employed. This evolution in computational chemistry, powered by quantum computing, is opening new frontiers and possibilities.
Quantum Computing and Chemistry: Key concepts
As mentioned, quantum computing represents a paradigm shift, utilizing the principles of quantum mechanics to revolutionize how we approach complex problems, particularly in chemistry.
Unlike traditional computing, which operates on a binary system of bits representing 0 or 1, quantum computing introduces the concept of qubits. These qubits can exist in a state of superposition, meaning they can represent both 0 and 1 simultaneously. This unique characteristic allows quantum computers to perform multiple calculations at once, vastly increasing their processing power.
Nevertheless, superposition is just one piece of the quantum puzzle. Entanglement, another quantum phenomenon, plays a role in enhancing computational efficiency. When qubits become entangled, the state of one qubit is directly correlated with the state of another, no matter the distance between them. This interconnectivity allows faster and more complex data processing, a crucial advantage in tackling intricate chemical problems.
Now, the hardware implementing these qubits varies, with several types being explored for their potential in quantum computing. Superconducting qubits, used by companies such as IBM, are popular due to their scalability and relatively advanced development stage.
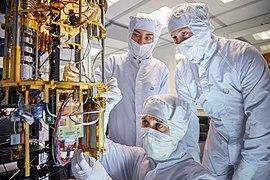
Image showcasing the cryogenic part of the quantum computer and coplanar resonators. © Wikimedia commons.
Quantum annealing, another approach to quantum computing, holds promise in chemistry. It involves gradually changing a system to reach its lowest energy state, which can be particularly useful in finding optimal solutions to complex problems. For example, a study from the Volkswagen Group and the Leiden University, demonstrated the use of quantum annealing to find the ground state energy. This study marked a significant step in applying quantum annealing to quantum chemistry, although it also highlighted the need for advancements to leverage its potential.
As quantum computing continues to evolve, its implications for the chemical industry are becoming increasingly evident. The ability to process massive amounts of data with unprecedented speed and accuracy opens new horizons in chemical research and development, promising breakthroughs that were once beyond reach.
Impact of Quantum Computing on Chemistry
As we transition from understanding the fundamentals of quantum computing, let’s explore the real-world applications in chemistry. Quantum computing’s unique capabilities are enhancing our understanding of complex chemical processes and accelerating advancements in drug discovery, material science, and optimization techniques. Let’s delve into these areas to appreciate the profound impact quantum computing is making in the chemical world!
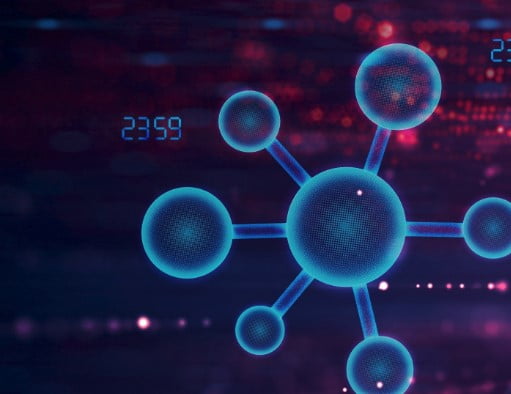
Drug Discovery and Biological Complexity
In drug discovery, quantum computing is opening new avenues for understanding biological and chemical complexities. The drug discovery process involves unraveling disease pathogenesis and identifying underlying mechanisms through advanced technologies like transcriptomic. However, the intricacy of these analyses often makes it challenging to pinpoint optimal drug targets. This is particularly true for complex disorders like Alzheimer’s, and multiple sclerosis.
The task of identifying the right molecule to bind to a discovered target is daunting, given the vast chemical universe comprising a huge number of organic compounds potentially suitable for therapeutic use. Quantum computing, in synergy with AI-powered solutions, is poised to revolutionize the efficiency of drug discovery. Companies across Europe, like Aquemia, Qubit Pharmaceuticals, and CreativeQuantum, are leveraging quantum technologies alongside AI to optimize drug research processes.
Advancements in Material Science and Nanotechnology
Quantum computing is also making significant strides in material science and nanotechnology. A notable example was a study done in Taiwan, which addresses the traditionally NP-complete problem of predicting a protein’s natural shape based on its sequence. This study demonstrates how quantum computational approaches can significantly expedite protein structure prediction, outperforming classical methods by a factor of four. Such advancements have far-reaching implications in not only chemistry, but also physics, bioinformatics, medicine, and quantum biology.
Optimization in Chemical Processes
In chemical processes, optimization often involves finding the best parameter combination among numerous variables. The challenge lies in the number of combinations to test to arrive at the most suitable solution. Quantum computing introduces a new level that allows for testing multiple combinations simultaneously, as opposed to the sequential approach of traditional computing. This capability, coupled with the immense computational power of quantum computers, enables quicker resolution of complex optimization problems.
These examples provide a glimpse into the possible contributions of quantum computing in chemistry. As we further explore the capabilities of quantum mechanics, it holds the promise of enhancing the scope and depth of chemical research and applications, opening new avenues for exploration and discovery.
More Real-World Application: Quantum Computing in Pharmaceutical Research
In the rapidly evolving landscape of quantum computing, its application in chemistry, particularly in pharmaceutical research, stands as a testament to its potential. A compelling example of this can be seen in the recent advancements made by Qubit Pharmaceuticals in November 2023. Their groundbreaking work exemplifies the practical application of quantum computing in addressing complex chemical challenges.
This study showcases the use of a Hyperion GPU-accelerated state-vector emulator for quantum state preparation (QSP) on the ground state of strongly correlated systems, utilizing up to 28 qubits. The essence of this approach lies in encoding a many-electron wave function, which typically scales exponentially, onto a qubit register that scales linearly. This method demonstrates a significant leap in overcoming the limitations of traditional quantum chemistry methods.
Qubit Pharmaceuticals’ innovative approach combines both classical and quantum computational methods. By initializing the qubits to a high-quality approximation of the desired ground state, they set the stage for efficient and effective ground state quantum algorithms.
This collaboration between quantum computing and pharmaceutical sectors highlights a growing trend in leveraging quantum technology for the acceleration of drug development processes. It reflects a broader movement in the market, where there’s an increasing demand for precise and efficient drug discovery, material design, and chemical simulations. These advancements are not just theoretical but have practical implications, evidenced by the investment from research institutes and government entities in quantum computing research.
Qubit Pharmaceuticals’ work is a clear example of how quantum computing is no longer a distant promise but a present reality, shaping the way we approach and solve complex problems in chemistry. Their commitment to exploring the interplay of classical and quantum methods opens new avenues in quantum chemistry simulations, underscoring the transformative role of quantum computing in various scientific domains.
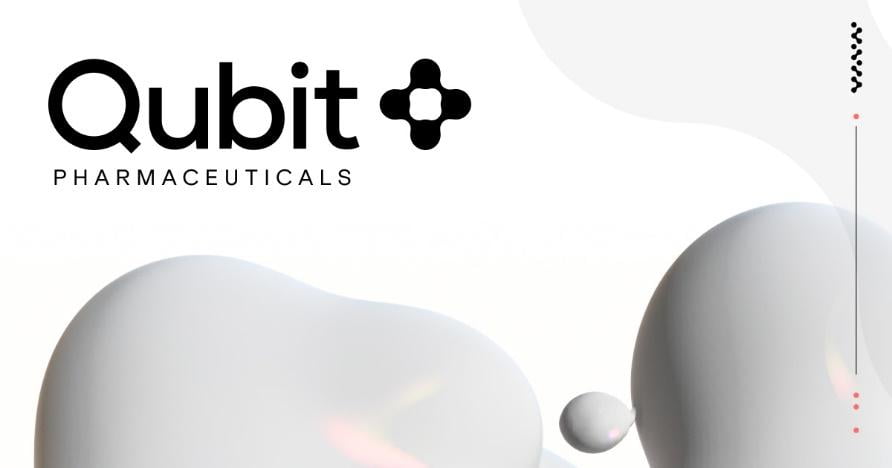
© Qubit Pharmaceuticals.
Navigating the Challenges: Realities of Quantum Computing in Chemistry
Quantum computing’s integration into the field of chemistry is not without its challenges. The road to commercialization and scaling of quantum computing applications involves navigating a landscape of hardware and software complexities. Before finishing, we will address the realities and hurdles in implementing quantum computing in chemical research and how advancements in chemistry are aiding in overcoming these obstacles.
Overcoming Hardware and Software Difficulties
The physical realization of qubits, the fundamental building blocks of quantum computing, is a subject of ongoing research and development. A significant challenge in this domain is the susceptibility of qubits to errors caused by environmental factors such as noise, vibrations, temperature fluctuations, and electromagnetic interference. These external disturbances can disrupt the delicate quantum state of the qubits, thus affecting the very essence of quantum computing. To combat these issues, quantum error correction methods are employed.
Chemistry’s Role in Developing Quantum Computing
Interestingly, the field of chemistry offers promising solutions to some of these challenges. A notable example is a 2022 study from the Università di Parma in Italy. This research explores the use of molecular spin systems as a platform for quantum computing, taking advantage of the ability to coherently manipulate multiple levels within single molecular structures. The approach suggests embedding quantum error correction within these molecular constructs, significantly simplifying its realization.
By integrating chemistry’s molecular-level precision with quantum computing’s advanced computational capabilities, this approach highlights a symbiotic relationship between the two fields. It exemplifies how advancements in chemistry not only benefit its field but also play a pivotal role in the evolution and refinement of quantum computing technologies.
Integrating quantum computing into chemistry presents complex challenges. But it is also defined by innovative solutions and collaborative efforts. As we continue to explore and refine these solutions, the fusion of quantum computing and chemistry holds the potential to unlock new scientific discoveries and technological advancements.
Conclusion
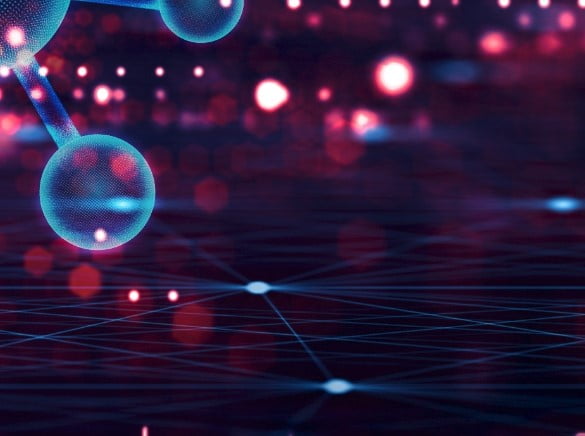
As we conclude this exploration of quantum computing’s role in chemistry, it’s evident that this cutting-edge technology harbors significant potential to reshape the field.
Key insights from our discussion highlight the practical applications and real-world examples of quantum computing in action. These instances not only illustrate the technology’s current capabilities but also point towards a future with possibilities. The synergy between quantum computing and chemistry is paving the way for more efficient, accurate, and groundbreaking scientific endeavors.
However, this journey is not without its challenges. As discussed, the intricate nature of quantum computing presents hurdles in hardware and software, requiring ongoing research and innovation. The field of chemistry itself plays a crucial role in overcoming these obstacles, offering solutions and collaborations that enhance quantum computing’s applicability and effectiveness.
Looking ahead, the prospects for quantum computing in chemistry are expansive. Quantum computing promises not just to transform the way we understand and manipulate the molecular level, but also to open doors to solutions for some of the complex and pressing challenges in chemistry today.
In summary, the intersection of quantum computing and chemistry is more than just a convergence of two fields; it is a burgeoning frontier of innovation, offering a glimpse into a future where the boundaries of scientific exploration continue to expand.