Imagine diving into a realm where the rules of reality are governed by principles that seemingly defy the very nature of existence. This is the world of quantum computing, where the qubit — the quantum bit — emerges as the cornerstone of a revolutionary computational architecture. Unlike the tangible, predictable nature of traditional bits, the quantum computing qubit ushers in a new paradigm where possibilities stretch beyond the binary constraints of 0 and 1.
The qubit definition pivots on its ability to embody multiple states simultaneously, thanks to a quantum quirk known as superposition. This unique trait empowers quantum computers to tackle complex problems at speeds unfathomable by their classical counterparts. In venturing through the basics of quantum computing qubits, we unlock an intellectual treasure chest brimming with potential, reshaping the future as we know it.
Key Takeaways
- Quantum computing qubits represent a pivotal shift from classical computing.
- Understanding qubits is crucial for grasping the power potential of quantum computing.
- The dual-state nature of qubits allows for complex problem-solving.
- Superposition is the essence of the quantum computing qubit’s capabilities.
- Quantum computing holds transformative prospects for a myriad of industries.
Introduction to Quantum Computing
Welcome to the intriguing and mystifying world of quantum computing—a frontier of technology that harnesses the principles of quantum mechanics. This is a domain where traditional computational rules are subverted, giving rise to exponential leaps in processing power and problem-solving abilities. At the core of this revolutionary approach is the quantum computing qubit, the fundamental unit of quantum information, which operates under rules defying classical physics.
The magic of quantum computing begins with quantum superposition, a principle allowing a qubit to exist in multiple states at once instead of being confined to the binary single-state existence of traditional bits. Meanwhile, the phenomenon of quantum entanglement adds another layer of complexity, permitting qubits to be interconnected such that the state of one can instantly affect another, regardless of the physical distance between them.
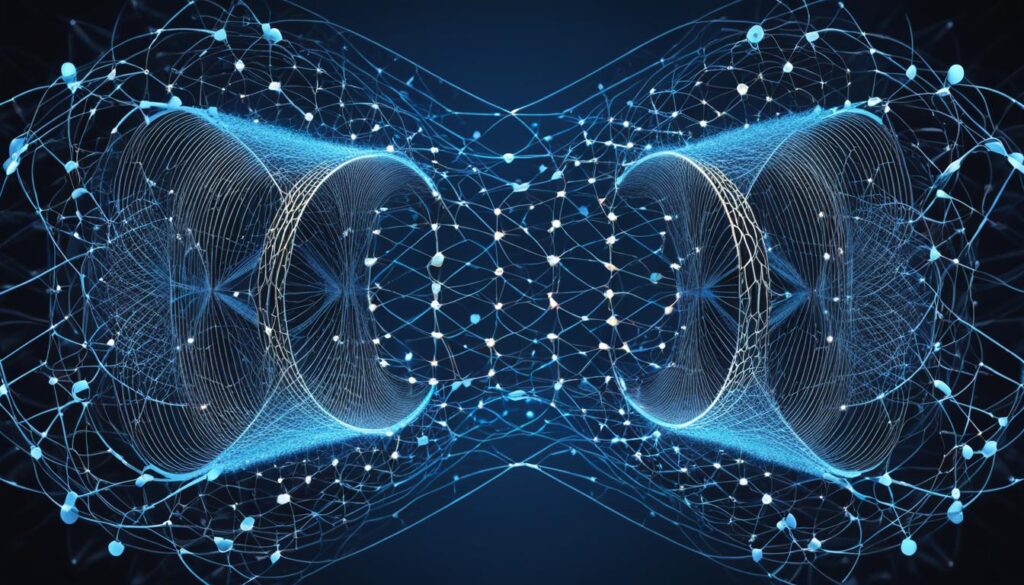
To truly appreciate the potential of quantum computing, imagine a ballet of qubits dancing in a complex choreography of superposition and entanglement. Through this elegant display, quantum computers can perform multiple calculations simultaneously, promising revolutionary advances in fields ranging from cryptography to materials science.
As we journey further into the understanding of quantum computing, we’ll dissect these phenomena to reveal how they work hand-in-hand to give quantum computers their awe-inspiring abilities.
“Quantum computing is not just a step forward in computing technology—it’s a leap into the realm of the nearly impossible, made possible through the delicate dance of qubits.”
With this foundation, we’ll be well-equipped to delve deeper into the essence of the quantum computing qubit and how it serves as the building block of potentially the most powerful computers our civilization has ever witnessed.
Understanding the Basics: Qubit vs Classical Bit
The stark contrast between the realms of classical computing and quantum computing can be traced back to their fundamental units of information: the classical bit and the quantum bit, or “qubit. When exploring the depths of classical computing, we identify the classical bit as the primary carrier of data, representing either a 0 or a 1 — a state of being that is fixed and unambiguous. This foundational aspect of classical computing has shaped technology as we know it, from the simplest calculators to supercomputers.
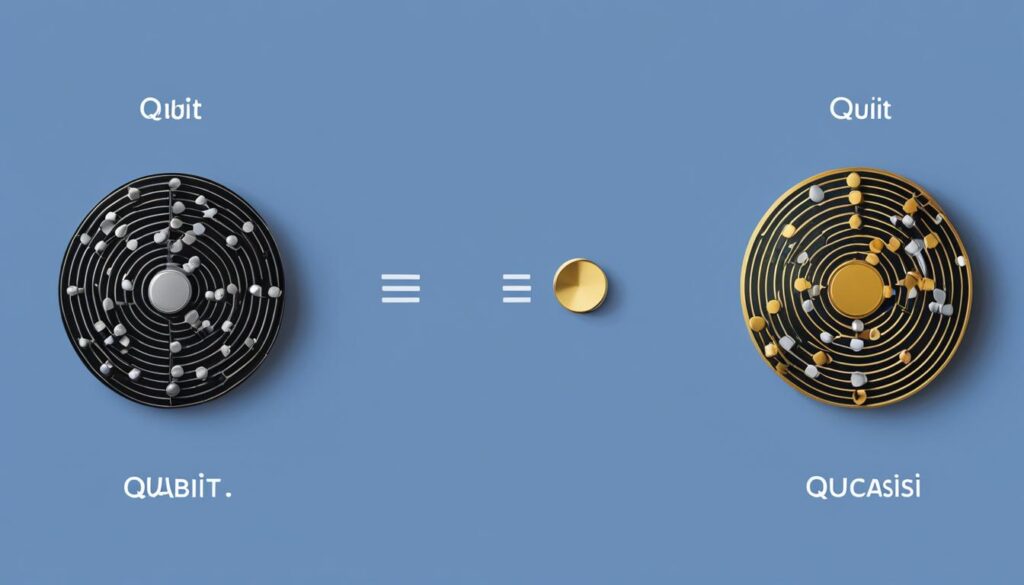
How Bits Function in Classical Computing
In classical computing, bits are analogous to switches that can be either off (0) or on (1). Every operation, no matter how complex, breaks down into a series of instructions that manipulate these bits in predictable, binary sequences. Data storage, data processing, and even internet communication rest upon the certainty of these binary states.
Differences Between Bits and Qubits
Transitioning our gaze to quantum computing, the qubit definition emerges as something more enigmatic. A qubit can simultaneously hold a 0, a 1, or any superposition of these states. This duality, a key distinction in the differences between bits and qubits, enables quantum computers to exist in multiple states at once, escalating their processing power significantly compared to their classical counterparts.
The quantum computing qubit extends beyond the binary to encapsulate the potential for multiple outcomes within a single operation. This inherent complexity of qubits allows quantum computers to process a vast number of possibilities concurrently, paving the way for solving intricate problems that are currently insurmountable by classical computers.
By understanding the core principles that differentiate the qubit from the classical bit, we unlock insights into a future where quantum computing transcends the boundaries of classical computing limitations.
The Quantum Computing Qubit Revealed
The allure of quantum computing lies in the element known as the quantum computing qubit. Fundamentally different from traditional bits, the qubit operates under the enchanting principles of quantum superposition and quantum entanglement. These foundational characteristics empower quantum computers with capabilities surpassing the realm of binaries to investigate solutions that classical computers find intractable.
In exploring the essence of quantum mechanics, we find that a qubit, being in a state of quantum superposition, can represent an infinite spectrum of values between 0 and 1. Rubbing the lamp of quantum computing, this superposition is the genie that grants the ability to process extensive computations in parallel rather than sequentially, as is the case with classical bits.
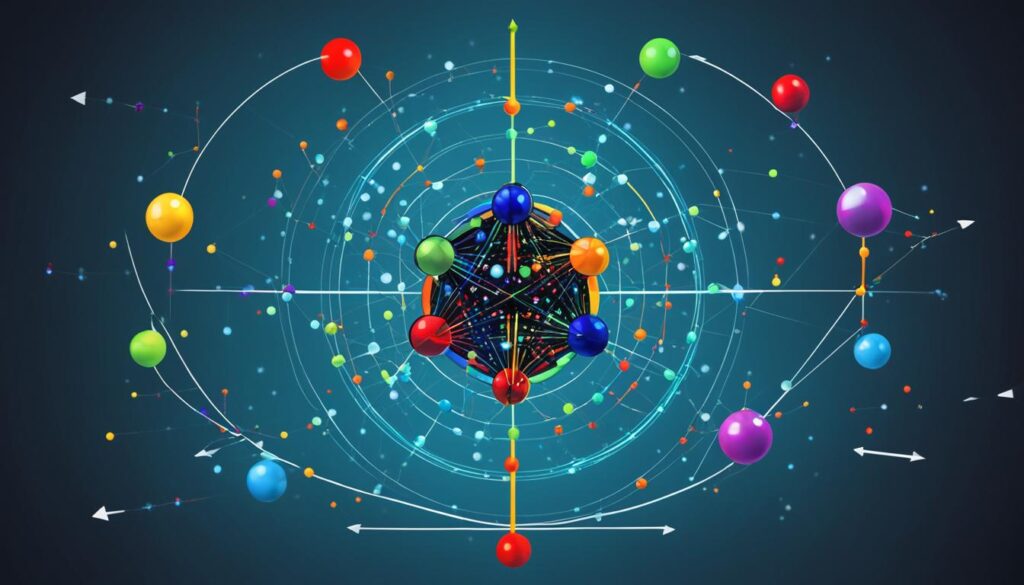
Moreover, quantum entanglement — another gem in the quantum crown — allows pairs or groups of qubits to be intertwined in such a way that the state of one, irrespective of distance, instantaneously influences the others. This mesmerizing connection adds another tier to the computational prowess that defines the next generation of high-speed problem-solving.
Feature | Classical Bit | Quantum Computing Qubit |
---|---|---|
States | Binary (0 or 1) | Superposition (0 and 1 simultaneously) |
Computational Power | Sequential Processing | Parallel Processing |
Entanglement Capabilities | None | Interconnected states |
Use Cases | Basic Computing | Advanced Problem-Solving |
The exploration into quantum computing qubit technology stretches the canvas of possibilities, painting scenarios that invite both awe and thoughtful consideration toward the computational challenges of tomorrow. As we continue to unravel the mysteries of quantum computing, these tiny powerhouses remain at the helm, guiding us through the quantum era.
“If classical bits enable us to glimpse the mountaintop, qubits promise to equip us with the rocket to reach its peak.”
Delving into Qubit Definition
The quest to comprehend the fundamental building blocks of quantum computing brings us to the very heart of the technology: quantum computing qubits. The qubit definition stretches our understanding of information processing by merging the classical concept of binary states with the multidimensional complexity of quantum mechanics. Qubits are not confined to the absolutes of 0 or 1—they thrive in a realm where they coexist as both, thanks to quantum superposition.
The Binary State of Qubits
Unlike traditional bits in classical computing that are resolute in their binary state, quantum computing qubits typify versatility and simultaneous existence. A qubit’s binary state is non-binary, creating a tapestry of possibilities woven by every quantum bit at play. This revolutionary attribute is what enables quantum computers to evaluate and process information on a scale that is unattainable with classical computers.
Handling Data with Quantum Bits
The process of handling data with quantum bits diverges from classical methods, embracing a phenomenon known as parallelism. This core feature allows for a swift, vast, and simultaneous evaluation of possibilities. Where classical computers solve tasks in a stepwise, linear progression, quantum computers, with their qubit-packed processors, perform calculations in concert, akin to an orchestra playing a symphony.
Aspect | Classical Bit | Quantum Bit (Qubit) |
---|---|---|
Representation | Single state (0 or 1) | Superposition (0 and 1 simultaneously) |
Processing | Sequential | Parallel |
Data Handling | Deterministic | Probabilistic |
Task Execution | One task at a time | Multiple tasks at once (Quantum parallelism) |
As we delve into the layered intricacies of a quantum computing qubit, we observe not just advancements in computational speed or efficiency, but a paradigm shift in what it means to process and understand data. The qubit, then, is the touchstone of a new era of computing, where myriad realities are reconciled within the microcosmic bounds of quantum states.
Quantum Superposition Explained
At the heart of quantum mechanics, and thus quantum computing, lies a fascinating concept: quantum superposition. This fundamental principle states that particles such as electrons or photons—and in the case of quantum computing, qubits—can exist in multiple states simultaneously. It’s a striking departure from the traditional binary states we’re accustomed to in classical computing, where bits are strictly zeros or ones.
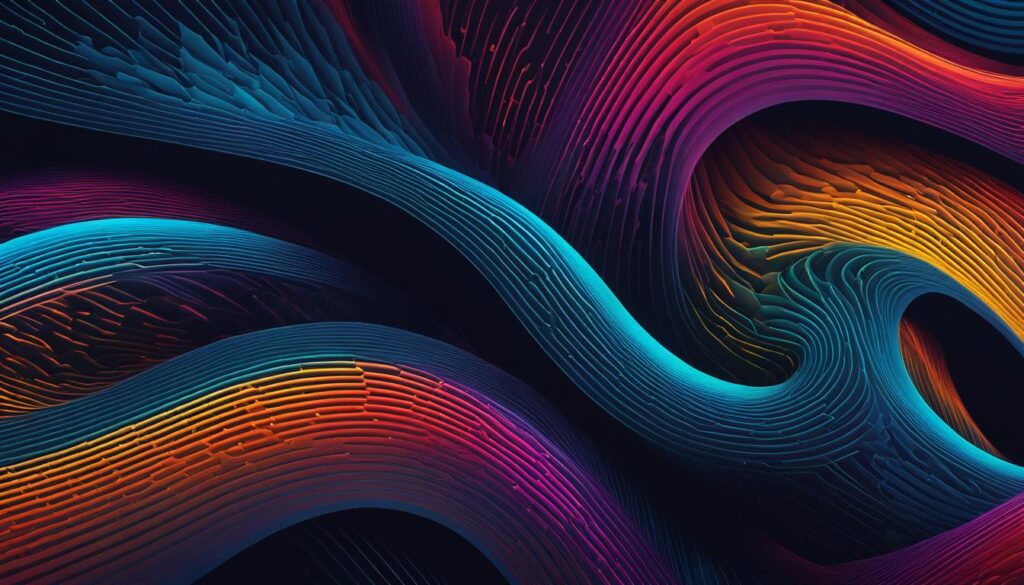
Superposition vs. Traditional Binary States
In classical computing, binary states are akin to a straightforward light switch with two clear positions: on and off. However, when we stroll into the quantum realm, things become less binary and more fluid. Superposition vs traditional binary states is like comparing the fixed state of a coin lying flat—heads or tails—with the myriad possibilities of its position while spinning mid-air. Quantum superposition allows qubits to hold a 0, a 1, or any quantum state in between, giving them the ability to encode and process a wealth of information with a single qubit.
Schrödinger’s Cat and Superposition
The paradigm of quantum superposition might seem abstract, so let’s simplify it with the famous thought experiment devised by physicist Erwin Schrödinger: Schrödinger’s cat. In this scenario, a cat in a closed box is simultaneously alive and dead—its actual state unknowable until observed. Similarly, a qubit holds various potential states at once but collapses into one upon measurement. This quantum quirk allows qubits to process complex calculations that would overwhelm conventional computers, setting the stage for extraordinary computational advancements.
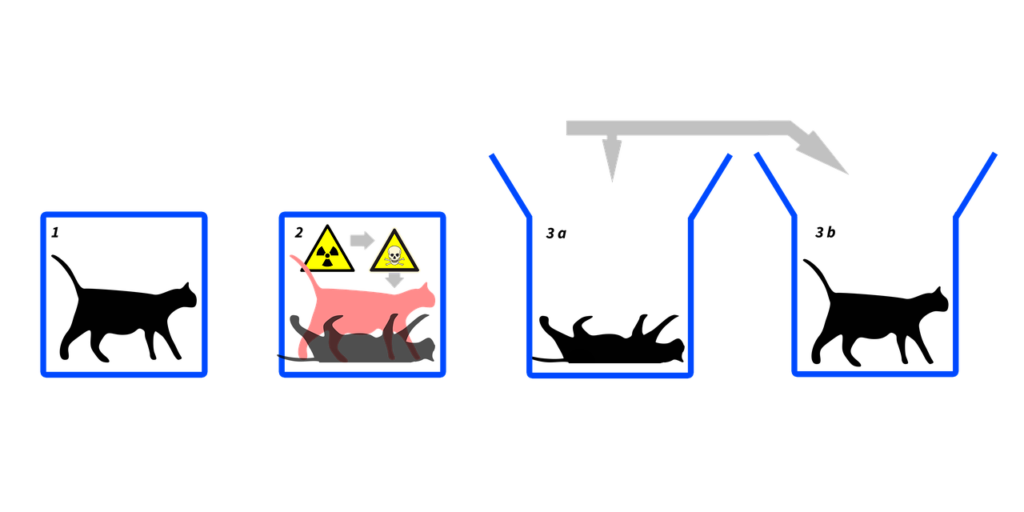
The intriguing contrast between quantum superposition and classical binary states is not only a novel concept but also the cornerstone of quantum computing’s promising future. As we grasp the profound implications of superposition, we also expand the horizons of what computers can achieve.
The Phenomenon of Quantum Entanglement
Delving deeper into the riveting world of quantum computing, we encounter the mysterious and enigmatic concept of quantum entanglement. This baffling phenomenon is fundamental to quantum mechanics, and by extension, paramount to understanding quantum computing and the qubit definition. But what exactly is quantum entanglement, and how does it function within the realm of quantum computing?
Quantum entanglement occurs when pairs or groups of particles interact in ways such that the quantum state of each particle cannot be described independently of the others, even when the particles are separated by a large distance. When we apply this to qubits, we understand that the state of one entangled qubit instantly correlates with its partner, creating a bridge that defies the limitations of space and time.
“Quantum entanglement weaves together the fates of qubits in a tapestry of correlation that stretches across the cosmos.”
Now, let’s break down the practical implications that this enchanting dance of particles has on the computing landscape:
- Entanglement enables quantum computers to perform operations at an exponentially faster rate compared to classical computers.
- The interconnected nature of entangled qubits is essential for complex algorithms that are beyond the capability of classical machines.
- It plays a vital role in quantum cryptography, ensuring robust security through the creation of unbreakable keys.
However, as we cast our sights onto the magic that is quantum entanglement, we also must consider the challenges it brings—a sensitive state that demands pinpoint precision to maintain. The subtleties involved in entangling qubits require delicate manipulation and control, making the realization of quantum computing a task for the brightest minds in physics and engineering.
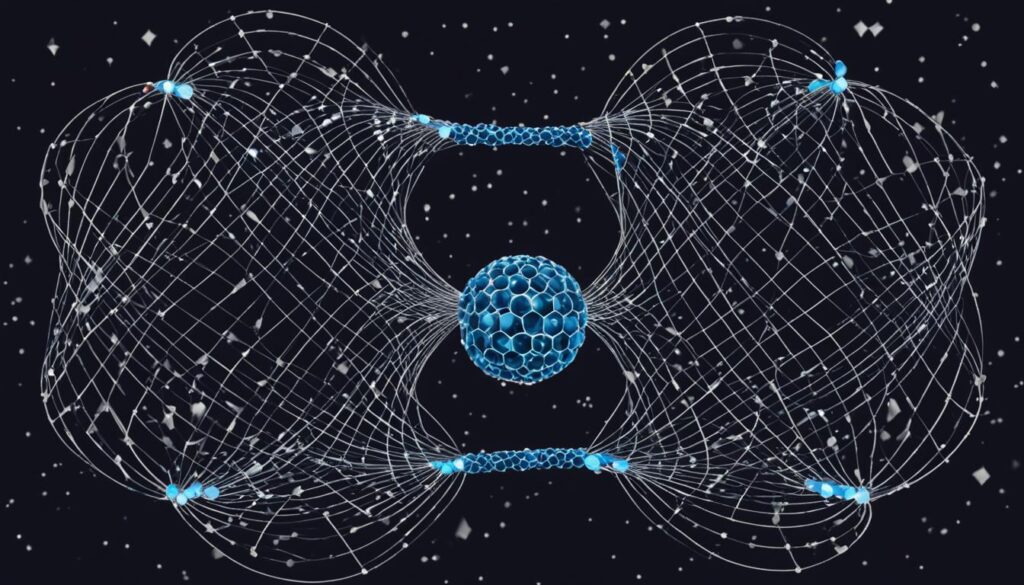
Attributes | Quantum Entanglement |
---|---|
Definition | A quantum state where two or more qubits are connected, no matter the distance between them. |
Key Feature | Correlation of states upon measurement. |
Importance in Quantum Computing | Allows complex operations and algorithms that surpass classical computing capabilities. |
Role in Cryptography | Enables the creation of highly secure communication channels. |
Quantum entanglement is not just a fascinating subject within quantum mechanics but also a pillar upon which the next generation of computing is being built. Understanding this curious intertwining of qubits is essential for grasping the full qubit definition and potential within quantum computing. It’s a quantum leap toward an era where our current technological constraints are but a distant memory, and the quantum realm offers solutions to challenges previously considered insurmountable.
Materializing the Concept: What is a Qubit Made Out Of?
The enigmatic qubit sits at the heart of quantum computing, intriguing with its ability to process complex mathematical problems with unprecedented speed. Yet, the question remains: What is a qubit made out of? In this section, we dive into the fascinating world of quantum mechanics to uncover the physical essence of qubits and the various materials being employed to actualize these quantum wonders.
Different Types of Qubits
Understanding qubits requires us to delve into the different types of qubits that researchers and engineers are developing. Each type offers unique advantages and represents the cutting edge in quantum computing technology. Some of the most promising qubits being explored today include:
- Superconducting Qubits: These are circuits made from materials that exhibit superconductivity at low temperatures, typically fabricated using aluminum or niobium.
- Trapped Ion Qubits: Utilizing atoms that are electrically charged, these qubits manipulate ions held in place by electromagnetic fields.
- Topological Qubits: Although still largely theoretical, these types of qubits promise high levels of stability by storing information in knots within the quantum state.
- Quantum Dot Qubits: Created within semiconductors, quantum dots hold electrons in a confined space, harnessing their spin or charge as the qubit.
- Photonic Qubits: Relying on the properties of light, photonic qubits use photons and are advantageous for quantum communication tasks.
The diversity among these types of qubits showcases the interdisciplinary effort that converges physics, materials science, and engineering to realize the full potential of quantum computing.
Real-world Materials for Constructing Qubits
Pushing the boundaries of our understanding of quantum mechanics, the search for real-world materials for constructing qubits is as intense as it is fascinating. The following are some of the tangible materials utilized in this pursuit:
Qubit Type | Material Used | Key Attribute |
---|---|---|
Superconducting Qubits | Aluminum, Niobium, Silicon | Low Resistance at Cold Temperatures |
Trapped Ion Qubits | Ytterbium, Calcium Ions | Control with Lasers and Electromagnetic Fields |
Topological Qubits | Exotic Materials (e.g., Majorana Fermions) | Robustness to Environmental Noise |
Quantum Dot Qubits | Gallium Arsenide, Silicon | Manipulation of Electron Spin or Charge |
Photonic Qubits | Silica, Silicon | Transmission and Encoding of Quantum Information via Light |
The innovation in materials science and nanotechnology has been pivotal in the progression from abstract quantum theories to applicable quantum devices. By exploiting the quantum mechanical properties of these different materials, researchers are witnessing real-world breakthroughs in quantum computing.
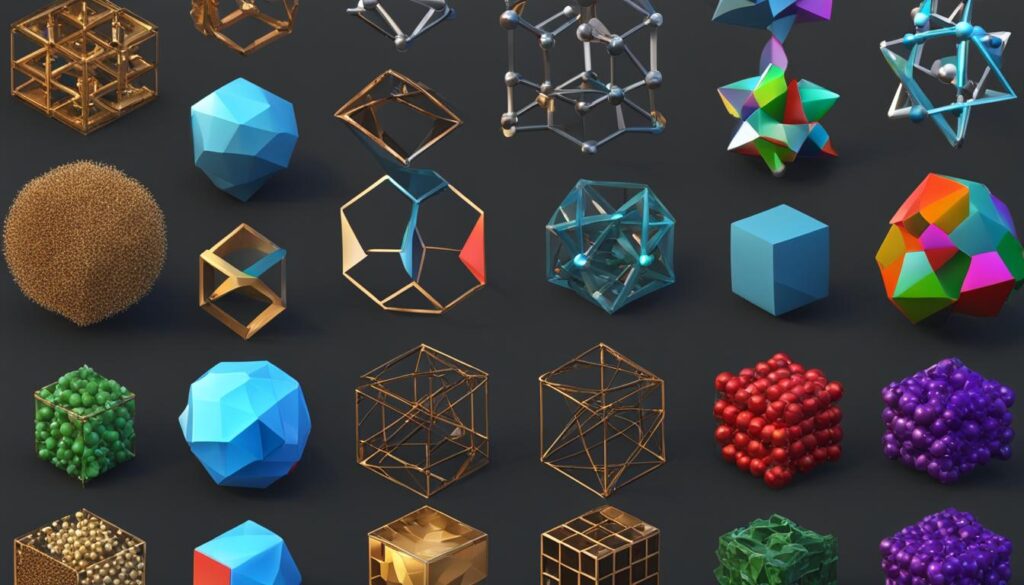
As we continue to navigate the intricate landscape of quantum computing, it’s apparent that the fusion of superior materials and innovative designs will drive the evolution of qubits. The fusion of these real-world materials for constructing qubits is not just a testament to human ingenuity but also a beacon for unprecedented advancements in technology.
Quantum Computing in Action: Use Cases and Applications
The dawn of quantum computing is upon us, bringing forth a revolution in the way we tackle the world’s most complex problems. Bolstered by the unique attributes of the quantum computing qubit, industries across the map—from healthcare to finance—are poised to be transformed. Let us embark on a journey through the fascinating landscape of quantum computing applications that are reshaping our world.
Quantum computing’s potential to crunch vast data sets and model complex systems carries implications that reach far and wide. In the realm of medicine, for instance, quantum computers are already showing promise in speeding up drug discovery and tailoring personalized treatment plans. In financial markets, their predictive power may revolutionize risk assessment and algorithmic trading by analyzing variables beyond the grasp of current computers.
Yet, the applications of quantum computing are not limited to these domains alone. The intersection of quantum mechanics and computer science has given rise to encrypted communications that are virtually impenetrable, addressing some of the most pressing security challenges in our digital age. In logistics and supply chain management, quantum computing could optimize routes and inventory systems, potentially saving billions and reducing environmental impact.
The unprecedented computational strength of quantum computing qubits is not just an incremental improvement, but a radical leap forward, enabling us to explore possibilties hitherto deemed impossible.
Consider the expansive table of use cases where quantum computing stands to leave its indelible mark:
Industry | Potential Quantum Computing Application | Impact |
---|---|---|
Healthcare | Drug discovery, molecular modeling | Faster breakthroughs in treatment |
Finance | Risk analysis, fraud detection | Enhanced predictive analytics |
Cryptography | Quantum encryption | Improved data security |
Energy | Optimization of grid distribution | Efficient resource management |
Material Science | Discovery of new materials | Innovation in technology and manufacturing |
The power of quantum computing also extends to the cosmos, as astrophysicists harness the power of qubits to simulate cosmic phenomena and unlock the secrets of our universe. The sophisticated simulations that are paramount to understanding dark matter and the quantum fabric of space-time are now within reach, opening new chapters in cosmic exploration.
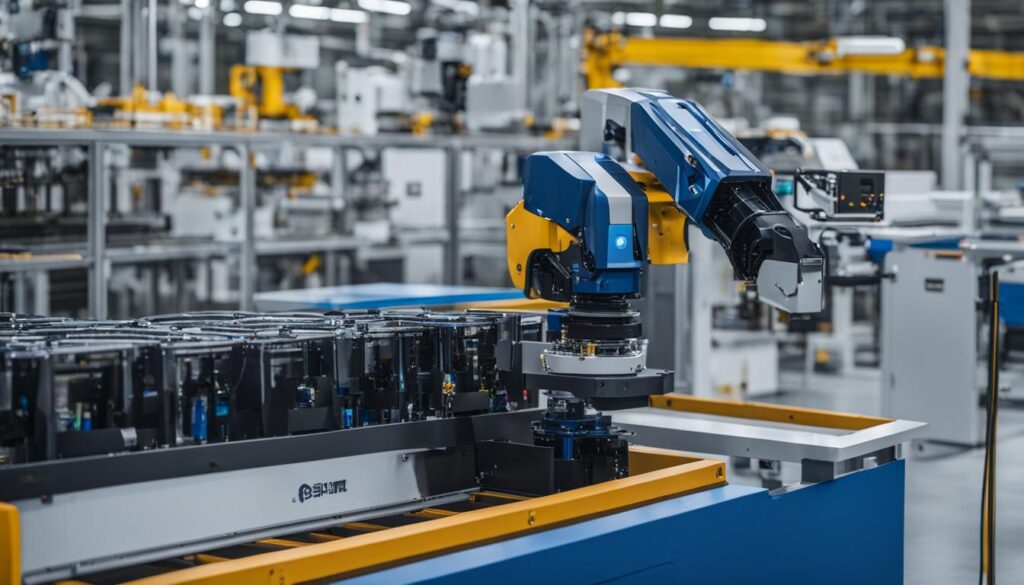
From climate science, where researchers can model complex environmental systems to predict changes, to logistics where quantum algorithms find the most efficient delivery paths, the fruits of quantum computing applications are vast. We stand on the cusp of a new era, where the quantum computing qubit is the beacon guiding us towards an uncharted future, rich with potential and pulsating with opportunities waiting to be realized.
Challenges and Limitations in Qubit Development
As we stand on the precipice of a new era in quantum computing, we must acknowledge the significant obstacles that loom on the horizon. One of the most critical aspects faced in the advancement of quantum systems is addressing the inherent challenges in qubit development. In this pursuit to harness quantum phenomena for computational prowess, two main hurdles stand out: the enigmatic issue of quantum decoherence and the formidable task of scaling quantum systems.
Decoherence: The Battle for Quantum Stability
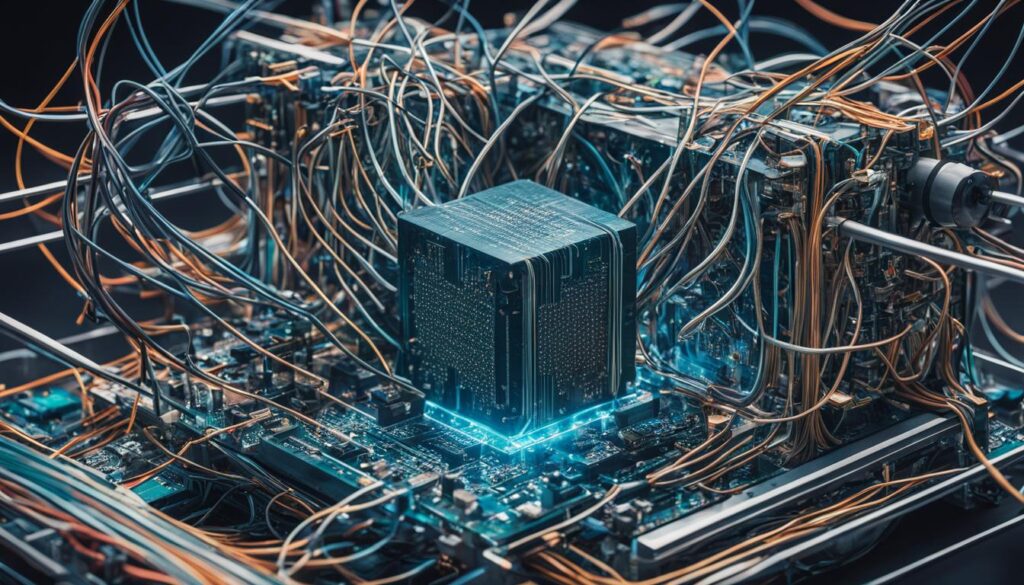
At the forefront of these challenges lies quantum decoherence, a formidable antagonist to the stability of qubits. As building blocks of quantum computing, qubits are delicate entities; their ability to maintain a coherent quantum state is paramount. Quantum decoherence represents the loss of this coherence, akin to a finely tuned instrument losing its harmony. The delicate balance of quantum superposition, where qubits exist in a blend of states, is susceptible to the slightest environmental interactions—from electromagnetic fields to temperature fluctuations—leading to a collapse of the superposition and a resultant loss in quantum information. This phenomenon poses one of the most significant qubit limitations, demanding innovative isolation and error-correction strategies to overcome the ephemeral nature of a qubit’s quantum state.
Scaling Quantum Systems: A Technical Challenge
Another hurdle is Scaling quantum systems. As the intricacy of quantum-based tasks grows, so does the necessity for larger quantum computers with more qubits. However, increasing the number of qubits while maintaining their individual and entangled states is not a mere matter of addition. It’s a technical conundrum seasoned with intricacies related to quantum mechanics and engineering. The difficulty in achieving this is not merely logistical, but also rooted in a need for progressive miniaturization, enhanced control of qubit interactions, and advanced error-correction protocols. The complexity of scaling is proportional to the number of qubits, turning what seems like a quantifiable problem into a multidimensional maze.
In summary, while the promise of quantum computing and its potential to revolutionize industries is immense, the path forward is strewn with scientific and technical challenges in qubit development. Navigating the obstacles of quantum decoherence and scaling quantum systems requires a symbiosis of rigorous research, innovative thinking, and tenacious problem-solving.
Future Perspectives: The Road Ahead for Quantum Qubits
As we venture further into the 21st century, the future of quantum qubits shines bright on the horizon of technological innovation. Researchers and engineers worldwide are forging pathways through the current limitations, heralding a futuristic portrait where the prowess of quantum computing advancements might transform our computational landscape.
The collective aspirations of the quantum community conjure visions of qubits operating with unparallel stability and scale. The goals are ambitious: to have arrays of qubits so robust and interconnected that they become the bedrock of quantum computing devices, accessible not only to scientists but also to industry and end-users. This is the journey from lab to life—a global stride where qubits will not just be a buzzword but foundational to problem-solving in real-world scenarios.
Progress in quantum computing often feels like a quantum leap rather than a measured stride, and with every leap come newfound challenges and potential solutions. As we look to the future of quantum qubits, there are several key advancements that are both hoped for and predicted:
- Dramatic improvements in error correction algorithms to ensure qubit accuracy over prolonged computations.
- Breakthroughs in material science yielding substrates and environments that enhance qubit longevity, potentially unlocking room-temperature quantum computing.
- Novel architectures that facilitate large-scale entanglement and quantum network integration.
- Strides in quantum software to harness the raw power of qubits for more widespread and diverse applications.
Moreover, there’s a tangible buzz amongst leading quantum researchers about the concept of ‘quantum supremacy’—a state where quantum computers can perform tasks that are currently impossible for classical computers. As these fields mature and these devices emerge from pure theory into tangible reality, we will likely witness an entirely new branch of computation taking root, where the future of quantum qubits is synonymous with unexplored possibilities.
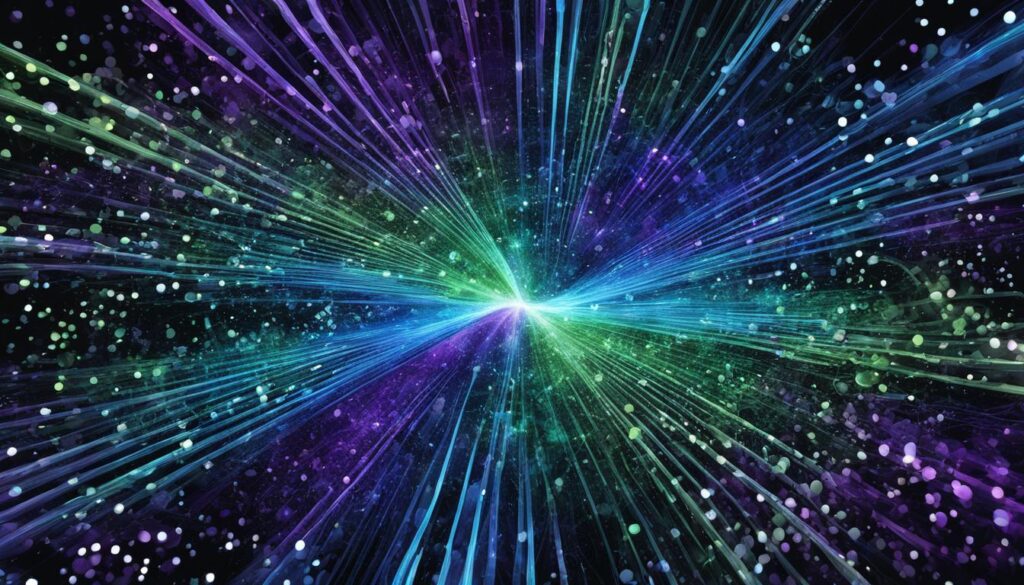
Let’s speculate further on the advancements that could enrich the tapestry of quantum computing:
Advancement Area | Potential Impact |
---|---|
Scalability | Greater number of qubits per chip leading to more powerful computations and diverse applications. |
Algorithm Development | Enhanced algorithms that capitalize on the characteristics of qubits, accelerating machine learning and optimization tasks. |
Cross-Disciplinary Integration | Blending quantum computation with other technologies like AI, resulting in smarter, faster decision-making systems. |
Economic Viability | Rising commercial quantum computers making this advanced computation a standard for industries. |
Quantum Networking | Secure quantum communication systems that could redefine data privacy and cybersecurity. |
The future of quantum qubits is not just an academic venture; it’s a bridge to an era of computing that will likely disrupt how we approach every task requiring calculation, analysis, prediction, and problem-solving. Amidst this whirlwind of incredible prospects, the scientific community, industries, and civil society alike teem with anticipation over the quantum computing advancements that loom just over the chronological horizon.
In these future musings, the essence of transformation lies not solely with the qubit and quantum mechanics—it is the very essence of human curiosity and ingenuity that propels us, hand in hand with these atomic-scale wonders, towards a future ripe with computational potential.
Conclusion
As we reflect on our journey through the intricacies of the quantum computing qubit, we find ourselves at an inflection point in technological progression. The voyage into the quantum realm has revealed a microcosmic universe where qubits challenge the very precepts of classical computing and beckon a future brimming with transformative potential. Summarizing quantum progress, we recognize the quantum computing qubit not merely as a curious entity but as the harbinger of groundbreaking change in computational power and efficiency.
Summarizing the Quantum Leap
The narrative arc of this exploration has outlined the key features of quantum computing—from the duality of superposition to the entanglement phenomenon—each underscoring the astonishing versatility of qubits. We have observed the tangible impact of quantum qubits across numerous industries, foreseeing their capability to untangle the most profound puzzles and accelerate solutions to complex challenges. This encapsulation leads us to acknowledge the quantum leap in computing that qubits potentially embody.
Envisioning the Future with Quantum Computing
As we stand on the cusp of what might be the most compelling epoch in computing, the future of quantum computing unfurls like the vast expanse of the universe—limitless and filled with the unknown. The potential that lies within quantum qubits is not just an incremental step but rather a colossal bound forward, one that promises to revolutionize our global infrastructure, optimize resources, and even decrypt the hidden language of the cosmos.
Ultimately, it’s the impact of quantum qubits that lends an air of anticipation to the field. Just as the quantum mechanics once baffled the brightest minds of the last century, the operationalization of quantum computing stands as the frontier ready for today’s visionaries to explore. Our shared optimism for this emergent technology mirrors the phenomenal prospects these atomic-scale marvels offer—a quantum future, inevitably reshaping the world as we know it.