Imagine a world where the power of quantum processing is no longer just a theoretical marvel but an everyday asset. Welcome to the era of ion trap quantum computing, where charged particles suspended by invisible forces perform computational feats that make traditional processors look like relics.
With trapped-ion systems spearheading this revolution, we’re not just crunching numbers; we’re orchestrating the dance of qubits in a symphony of superposition and entanglement.
Unlocking the potential of quantum processing power involves harnessing the peculiar charm of the quantum world, where particles are both here and there, and existing in multiple states is just another day at the office. In this realm, trapped ions are the venerated workhorses, carrying the mantle of quantum information with fines precision and unhindered by the binary constraints of classical computing.
The allure of these systems lies in their extensibility. Building a scalable quantum computer is no walk in the park, but trapped-ion technology whispers promises of grand scale. It might sound like a quantum leap, but with each ion we entangle, we inch closer to unraveling the mysteries folded within unfathomably complex computations.
So buckle up, because ion trap quantum computing is not just a game-changer; it’s the game itself. And in this game, we’re playing for the power to pioneer untold innovations across every field imaginable. Ready to tap into the arcane arts of ions and qubits? Let’s dive into the quantum tide.
Key Takeaways
- Ion trap quantum computing holds the key to extraordinary computational abilities beyond classical limitations.
- Trapped-ion systems utilize the unique quantum mechanics of superposition and entanglement to create powerful quantum processing power.
- Advancements in ion trapping techniques are nudging us closer to the dream of building a fully scalable quantum computer.
- Combining electromagnetic wizardry with precise laser techniques, scientists are entangling ions with unprecedented accuracy.
- The field of quantum computing is not only transforming our computational capacity but is also driving innovation in materials science and integrated technologies.
- With scalability in sight, the future of ion trap quantum computing looks brighter than ever.
The Basics of Ion Trap Quantum Computing Technology
Delving into the heart of ion trap quantum computing, we uncover a realm where quantum information is no mere abstraction but an electrifying reality.
At the crux of this technology, electromagnetic fields coax ions into a high-tech dance, conspiring to confine them without the touch of any physical container. Yes, it’s the modern-day wizardry of ion trapping, and it’s putting classical computing in the rear-view mirror.
Behold the mighty Paul trap, the Nobel Prize-winning innovation by Wolfgang Paul, which forms the nucleus of this avant-garde pursuit. Through an oscillating electrical waltz, this trap choreographs ions into a sedentary linear conga-line. With ions now stage-ready, cue the lasers.
Ion Trapping Techniques and Qubits
Plucked from the pages of a sci-fi novel, ion trapping techniques are a tale of controlled cosmos within a chip. Here, qubits—quantum’s answer to binary bits—are the protagonists in quantum computing’s narrative. But these aren’t just any qubits; they’re the cool custodians of electronic states, immutable in their resolve to store and process data beyond the binary.
Laser-Induced Coupling for Quantum Operations
A laser here, an entanglement there—a simple flourish of laser-induced coupling, and voilà, single qubit operations unfold with the finesse of a maestro’s baton. This intricate pas de deux between photon and ion is the secret sauce to enacting quantum operations that are as precise as they are revolutionary.
Scaling Ion Trap Architectures for Quantum Computing
The quest for a scalable quantum computer is akin to building Rome—patience and ingenuity are key. Enter ion trap architectures, evolutions in design thinking that suggest—wait for it—qubits can take the scenic route around quantum charge-coupled device (QCCD) arrays without forgetting where they started. Imagine, a day when “ion trap on a chip” isn’t just technobabble, but the standard.
As we stand on the cusp of great computational dominion, we realize that the journey to a fully-functioning scalable quantum computer is not a sprint; it’s a marathon with quantum hurdles. Yet, with every cooled ion and synchronized laser pulse, we inch ever closer to conquering the quantum realm. Onward, to the quantum odyssey!
Pioneering Steps of Ion Trap Quantum Computing
When Cirac and Zoller brainstormed a quantum conundrum back in 1995, little did they know that they were etching the cornerstone of trapped-ion quantum computing. This was no mere thought experiment; it was a vision that would galvanize the whole field into action. With the steering contribution of the NIST Ion Storage Group, the differential dreams of quantum computing began turning into kinetic reality. Fastening your seat belts is advised—this journey through pioneering quantum escapades is not for the faint-hearted.
The Ground-Breaking Work of Cirac and Zoller
As true pioneers, Cirac and Zoller tossed the classical blueprint out the window to conjure the controlled-NOT quantum gate, an elemental piece of quantum bravura. Their landmark proposal didn’t just introduce a new character to the quantum narrative; it reshaped the story arc for entangled states and precision-controlled quantum operations.
Major Milestones in Trapped-Ion Experiments
Each trapped-ion experiment enriches our quantum vocabulary, contributing terms like “superposition,” “coherence,” and “quantum computer accuracy.” From fine-tuning the nuances of entangled ion chains to choreographing ions with laser precision, these milestones are bursting the boundaries of what was perceived to be quantumly possible.
Innsbruck’s Compact Quantum Computing Demonstrator
And then came 2021, the year when Innsbruck’s whiz minds unveiled not just a quantum computer, but a compact trapped-ion quantum computing marvel. Crisp and cozy in its dual server racks, this progeny of Innsbruck is the poster child for scalable quantum computers—and quite rightfully so.
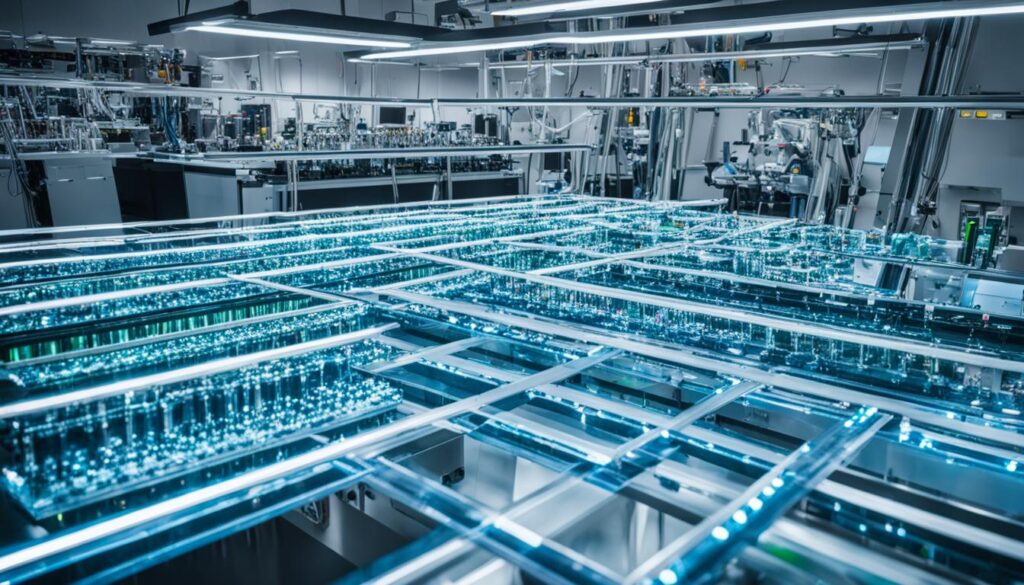
Like threading a needle in a quantum storm, aligning ions in a server rack-sized space and maintaining high fidelity is no trivial pursuit. Yet, in its own stylish rack mounts, Innsbruck’s demonstrator couldn’t exude a cooler “quantum chic.” This compact approach tantalizes with promises of quantum computing at scale, ushering an age of accessible entanglements and qubit cadenzas echoing down the digital hallways.
- The blueprint for scalable quantum computers that Cirac and Zoller rolled out in ’95: still the reference manual.
- The NIST Ion Storage Group: Where qubits dare.
- Trapped-ion experiments sparking more intricate entangled states with each endeavor.
- Quantum computer accuracy: not just a measure, but the measure.
- Does size matter? Ask Innsbruck’s two-rack compact trapped-ion quantum computing achievement.
And so, our brushstrokes have sketched a bygone quantum daydream now bursting into a vividly detailed panorama. Cirac and Zoller’s controlled-NOT gate was but the first curve of entanglement; the real masterpiece is what comes after. In a haze of glowing ions and bulletins heralding the onset of Innsbruck’s quantum computing contributions, the narrative moves ever forward—toward a quantum epoch where “impossible” is a term bereft of meaning.
Understanding the Paul Trap
Step into the quantum arena where Wolfgang Paul, a virtuoso in the symphony of physics, composed the groundbreaking electrodynamic quadrupole ion trap. It’s no high-brow historical footnote; Paul’s trap is a cornerstone of our quantum computing renaissance. In the 1950s—when tailfins were all the rage, and computers filled rooms—the future Nobel Prize laureate envisioned particles dancing to the rhythm of an oscillating electric field, paving the way for quantum leaps in processing power.
“We confine the invisible, to compute the impossible” – a mantra befitting Wolfgang Paul’s seminal contribution to quantum mechanics, encapsulated by his invention, the Paul trap.
This ingenious device is the gravitational center pulling charged particles into orbit. Metaphorically, of course, as we’re talking electric potential, not gravity. It’s a place where ions cozy up at their energy saddle point, tethered by an invisible lasso of electric fields oscillating with just the right frequency to keep these charged cowboys corralled. And within these traps, ions are more than mere captives; they are the qubits, the elemental bits of quantum computing, holding secrets of unfathomable calculations in suspended animation.
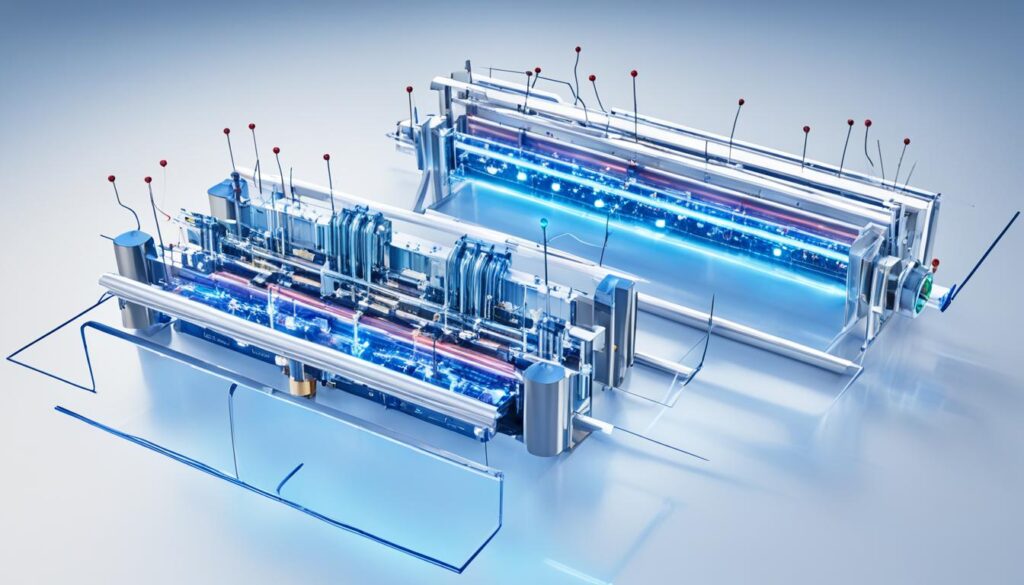
Component | Function | Significance in Quantum Computing |
---|---|---|
Oscillating Electric Fields | Create the dynamic potential well | Traps ions for use as qubits |
Radio Frequency (RF) | Determines the trapping conditions | Enables fine-tuning of ion positions |
Saddle Point | Lowest energy point within the trap | Defines the stable position for ion qubits |
Mathieu Equations | Describe ion motion within the trap | Crucial for predicting and controlling qubit dynamics |
So, we stand on the shoulders of Wolfgang Paul, staring out over a quantum landscape brought into view by his Nobel Prize-scooping insight. Today, the Paul trap isn’t just a neat lab trick; it’s the spectral hand that cradles the building blocks of tomorrow’s quantum processors, the electrodynamic quadrupole ion trap undeniably shaping the future as we know it.
Alas, with every quantum bit of information, we owe a tip of the hat to Wolfgang Paul, the pioneer who trapped potential in a quantum well—both literally and figuratively.
Ion Trap Quantum Computing Components and Operations
Descend into the quantum vortex where two types of qubits reign as minuscule monarchs of computation: hyper-fine qubits and optical qubits. These pillars of trapped-ion quantum computing relish in their microscopic throne room—an ion trap system, where reigns of laser light crown them the rulers of qubit stability.
Hyper-Fine and Optical Qubits
In the majestic realm of quantum computation, hyper-fine qubits don the crown jewels of longevity, reigning supreme for what might as well be eternity in a superpositioned state. Meanwhile, their courtly brethren, the optical qubits, flaunt less stability but still command a respectable presence, far beyond the fleeting flickers of logic gate operations. It’s this royal duality that gives rise to the nuanced governance of ionic qubit states, each playing a pivotal role in the royal court of quantum computation.
Optical Pumping and Initial State Preparation
The ceremonial tradition of optical pumping heralds the ascension of an ion to its throne, appointing it to a quantum state with the precision and pomp befitting a sovereign of the nano-realm. This initial state preparation is the kingdom’s proclamation, declaring the ion trap system ready for the forthcoming saga of quantum computation, with all subjects—the ions—in harmony, awaiting the command to entangle and compute.
The Measurement Process in Ion Trap Systems
When the time comes to decree the state of the kingdom’s qubits, a spectacle unfolds, known as the qubit measurement process. A laser, much like the scepter of power, initiates a royal decree, coaxing forth a cascade of photon release with the aid of state detection techniques. The multitude of photons, valiantly emitted in this optical pageantry, are gallantly captured and counted, unveiling the true state of each qubit’s allegiance in the delicate dance of quantum computation.
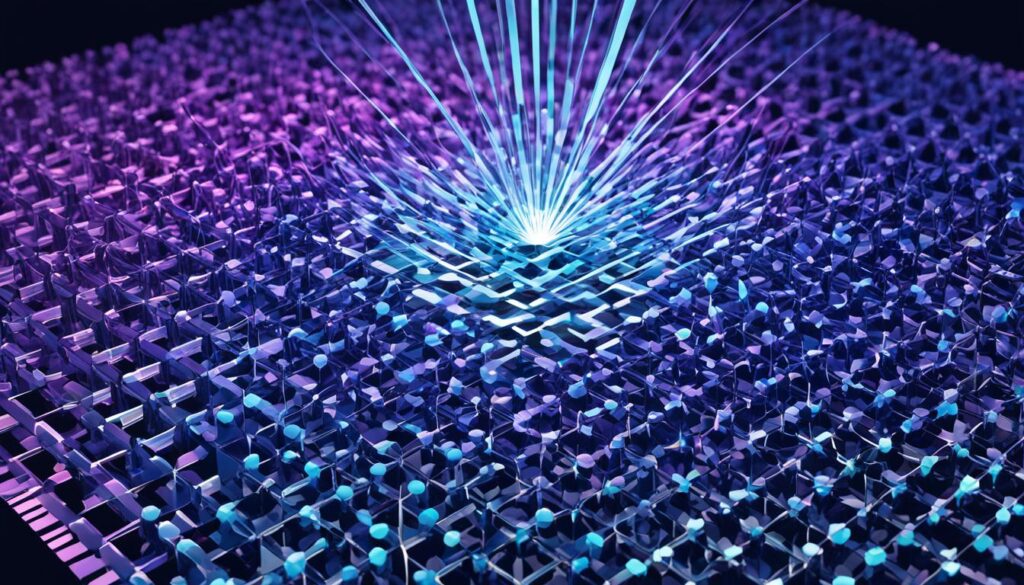
Through the looking glass of sophisticated instruments, this kaleidoscope of light unfurls the quantum narrative, with a PMT or CCD camera serving as the ultimate arbiter of state detection, decreeing whether a qubit has settled on ‘1’ or ‘0’. It is here that trapped-ion quantum computing transcends the physical, mapping the previously indescribable to the parchment of classical understanding—bit by entangled bit.
Advanced Techniques in Ion Trap Quantum Computing
The march towards the zenith of advanced quantum computing is underscored by a suite of innovative juggernauts pushing the ceiling of what’s attainable. Indeed, with the evolution of entangling gates, we glimpse the sublime craft of quantum machinations advancing towards grandeur. In this venture, the robust quantum gates that commandeer these operations are not merely components; they are conduits carving the path toward fault-tolerant quantum computation.
Take the vaunted entangling gates, for instance, which have become substantially more durable. Like the steadfast pillars of a stately bridge, they undergird the multi-dimensional computational acrobatics demanded by large-scale, interconnected quantum systems. We’re not just flirting with speed here; we’re courting velocity as we veer into the impulsive regime, a territory where gate operations could sashay sub-microsecond timescales, though such a marvel is yet to step out of theory’s shadow into the empirical daylight.
The realm of quantum gates extends beyond mere expediency, diving deep into the fidelity’s waters. Here, figures north of 99% are not uncommon, testimony to the stratospheric standard set for contemporary quantum endeavors. They are emblems of the relentless pursuit of a fault-tolerant dynast revered in quantum circuits—a testament to the daring lengths to which science dares to soar.
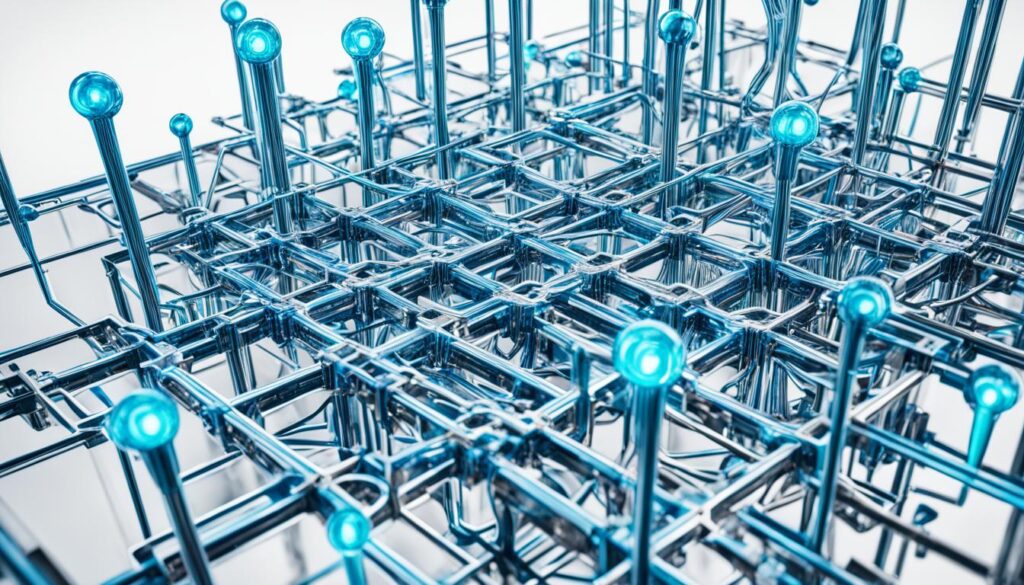
And as if the tales spun thus far weren’t sumptuous enough to satiate the intellectual palate, the narrative takes a gripping swerve. Researchers championing advanced quantum computing are not mere narrators of this quantum odyssey; they are the cartographers sketching the outlines of future landscapes. With each robust quantum gate minted and every protocol fortified, they arduously etch closer to the holy grail of computing: the prowess to perform the fault-tolerant tango within the quantum ballroom.
Advancement Area | Characteristics | Impact on Quantum Computing |
---|---|---|
Robust Entangling Gates | Increased durability and fidelity | Enables multi-particle qubit operations essential for complex algorithms |
Speed of Gate Operations | Theoretical feasibility of sub-microsecond operations | May dramatically reduce operation time, pushing efficiency |
Fidelity of Quantum Gates | Fidelity often exceeding 99% | Crucial for maintaining coherence and realizing fault-tolerant systems |
In sum, what is etched in the annals of quantum computing is nothing short of a saga—a tale that continues to unfurl its chapters with each pulsating beat of entangled ions. Through the lens of these advanced techniques and with eyes affixed on the horizon’s sheen, we embark further into the cosmos of possibilities, eagerly anticipating the next quantum leap.
Challenges and Innovations in Scalability and Integration
As we march towards quantum ascendancy, scaling the sheer cliffs of complexity presents both grand challenges and ingenious innovations. Like intrepid explorers, today’s quantum architects are weaving a logic lattice with interconnected traps expanding into larger qubit arrays. This intricate intertwining is not just about adding more qubits; it’s the art of maintaining their quantum coherence during the intricate ballet of ion transportation—a prerequisite for quantum algorithms to solve unsolved riddles.
Interconnecting Traps for Larger Qubit Arrays
The dream of a large-scale quantum solver entails a knight’s move in chess—strategic, bold, and with the potential to change the game. It’s the ion trap scalability that takes centerstage. Trapped-ion technologists have been conjuring designs where ions can tiptoe between traps, leap over electric-field noise, and bring forth a new dawn of computational possibilities, without spilling the cup of their coupled quantum state.
Emerging Materials and Technology for Noise Reduction
In the chamber of secrets where future quantum systems are being forged, new materials with enchanted properties are emerging. These materials are the silencers of the pervasive hum of electric-field noise. They are the alchemists’ stones tasked with transmuting distractions into coherence. By integrating cutting-edge materials, scientists aim to fine-tune ion-qubit control and, in doing so, compose the harmonics of high-fidelity quantum processing.
Integrated Quantum Systems: A Materials Perspective
From the materials perspective, every atom, every charge, sings a song of synergy. The melding of advanced materials into a single confluence of integrated quantum systems has the makings of an epic. It speaks to an era forged through collaboration between materialists and mystics of the quantum realm, a call to arms for both materials scientists and trapped-ion technologists to conjure a reality ripe with computational potency.
With a crescendo of innovation, the integration of materials and components for quantum systems rings across the quantum field. It’s a duet that beckons a future where the term ‘scalability’ isn’t followed by a forlorn sigh, but by a confident nod, affirming the arrival of practicable, large-scale, and dare we say, supreme quantum computing.
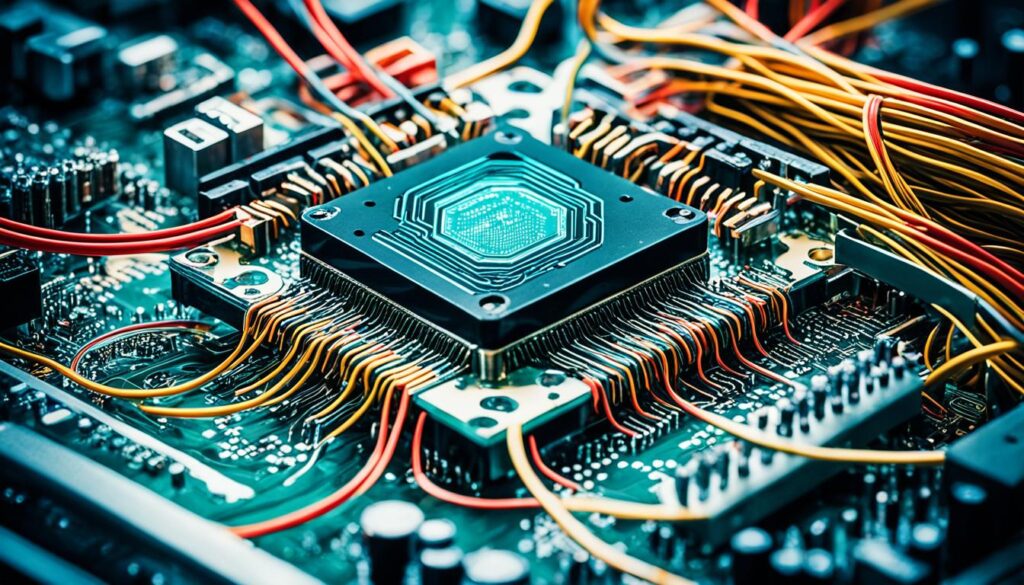
Innovation | Material/Component | Battle Against Noise | Integration Success |
---|---|---|---|
Ion Trap Design | Emerging Nanofabrics | Minimize electric-field noise | Facilitates complex qubit architectures |
Material Sciences | New Conductive Alloys | Reduce systemic decoherence | Enhances ion-qubit control |
System Components | Integrated Optics & Electronics | Diminishes extraneous interactions | Simplifies quantum operations |
Indeed, as we geniusly galvanize our efforts towards a materials perspective that can seamlessly bind with quantum alchemy, the ivory towers of current computational limits seem not so insurmountable. With the perfect potion of system components integration designed by the hands of wizards and custodians of quantum domain, the story of ion trap quantum computing continues—sprightly stepping into realms once whispered as legend.
Conclusion
As we weave together the intricate tapestry of quantum advancement, trapped-ion quantum computers emerge as the harbingers of a new computational dawn. With their innate ability to juggle the quantum realm with high precision and the beckoning horizon of scalability, these systems ink the prelude to a versatile quantum computer. Where classical computing tiptoes, practical quantum computation leaps bounds, driven by the relentless innovations of pioneers infusing life into the notion of a robust quantum future.
The mettle of this promising venture is fortified by the melding of genius minds in materials integration, a pivotal movement in overcoming the noise and quibbles of yesteryears’ attempts. The symphony of these materials working in lockstep with cutting-edge quantum components endows these computing maestros with the potential to deliver unparalleled computational power. It’s not just a step; it’s a quantum stride towards dominating the once-elusive realm of quantum supremacy.
The future of quantum computing gleams with promise as collaboration across disciplines sharpens the edge of what’s possible. Together, materials science and quantum technology are the torchbearers of progress, weaving a narrative rich with potential and filled with the anticipation of what quantum tomorrows may bring. For on the rippling edges of this frontier lies the heart of a revolution—a world where quantum computers shape realities once nestled in the haven of imagination.