Quantity of Qubit vs Performance
As techies and techpreneurs make efforts to grasp the finer points of quantum computing (QC) technology, they often ponder over the relationship between the qubit and the performance of the next-gen technology.
Come to think of it, because qubit is a basic unit of quantum information, one would expect that increasing qubits will automatically translate to revving up the performance of QC systems. Although that assertion holds true to a large extent, you have to consider certain caveats or crucial factors.
So, this article will dissect all these factors in detail and conclude with a satisfactory answer to the question: Does Increasing Qubits Improve Quantum Computing Performance.
Modality (Model)
Beyond enhancing qubits, modality (or simply model) affects the performance of QC systems. As you are aware, there are two major models used in tackling issues in QC technology, namely the gate-based model and quantum annealing. Quantum annealing focuses on optimizing the solution to problems, especially if there are several options for tackling them.
This means that the moment the “good enough” or “local minima” is known, the solution becomes easy to speed up. The downside of this model is that it cannot effectively run Shor’s algorithm, which shatters the modern cryptography used for protecting bank information, logins, web communications, etc.
On the other hand, a universal gate model relies on incrementally building reliable qubits to run complex algorithms. Over 50 algorithms, including Shor’s and Grover’s algorithms, run on this model. In other words, the universal gate-based model can be used to solve more issues than the quantum annealing model.
According to Google, D-Wave has developed a quantum annealing system 108 times faster than the regular computer chip. Keep in mind though that the qubits of quantum annealers are much easier to increase than those of gate-based QC systems because unlike the former which focuses on solving optimization problems, the later can run several complex algorithms and solve multiple problems. In simple English, rocketing the qubits of quantum annealing systems does not make them solve the complexities of puzzles that the gated model can.
Quantum Volume
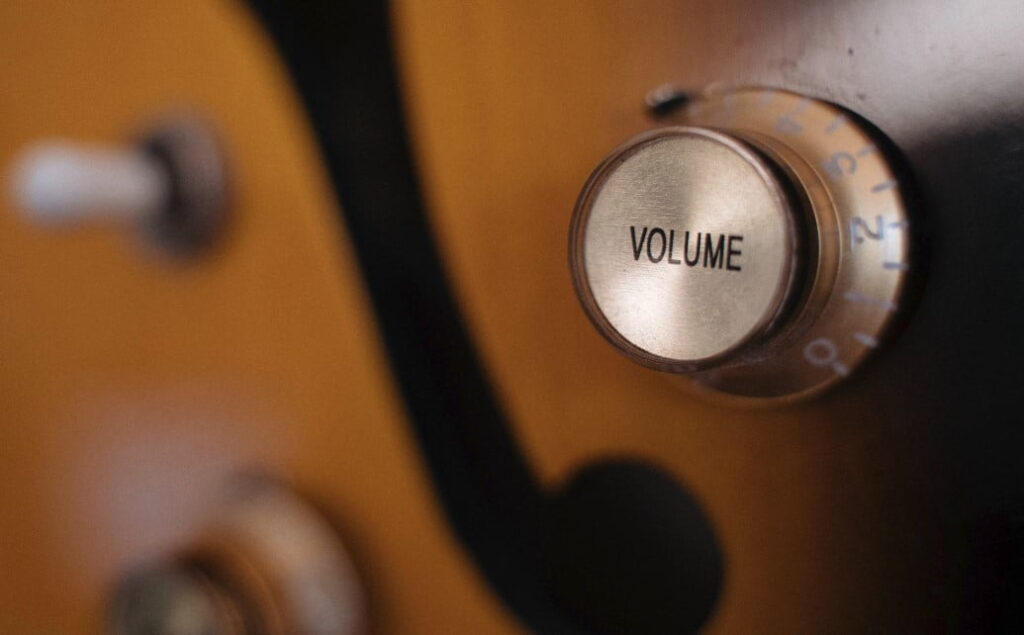
The brainchild of IBM engineers, quantum volume explains the various factors that contribute to the performance of gate-based QC systems. It is the measure of the QC system’s overall power and performance. Once the quantum volume’s numerical value is known, it becomes pretty easy to determine the kind of puzzle the machine can solve.
The number of qubits and operations that a QC system can solve is known as the width and depth of its quantum circuit. As the value of a quantum circuit increases so does its ability to run complex algorithms. But then, that’s not all. Number of qubits, gate and measurement errors, cross talk, efficiency, and the layout of connection among qubits also affect circuit depth.
Furthermore, you can say that the quantum volume analyzes the efficiency of those aforementioned parameters and produces a single number used to determine the system’s efficiency. The larger the value, the more powerful the machine becomes.
Relatively high error rates, crosstalk and short coherence time (as you will learn later) are some of the obstacles in today’s QC systems because they are barriers to building scalable and reliable QC systems. Years of studies show that reaching quantum advantage by 2025 will require designing and developing machines with a quantum volume of 1000 or more.
QC technology is still in its development stage. However, with the in-depth knowledge of quantum volume, researchers can further their studies, draw up a roadmap and optimize the architectures needed to advance the disruptive technology.
Coherence Time
Now, let’s look at the effect of coherence time on the performance of QC technology. First of all, coherence time is the length of time information can be held and manipulated. The longer quantum coherence, the richer and more interesting the applications get. Decoherence, on the other hand, occurs due to noise, leakage, and decay channels.
An Israeli quantum physicist, Itsik Cohen, has carried out extensive research into coherence. In his findings, Cohen posits that for you to maintain coherence in the system, waves must preserve their relative phase because doing so enables interference to take place.
Another factor that affects coherence is the ability to maintain superposition (of phase and amplitude). If superposition is kept stable, it becomes easy to maintain coherence.
Plus, if QC systems exhibit much longer coherence, the outcome is that it enhances their performance and higher operation fidelity. As stated earlier, the primary causes of decoherence are noise, leakage, and decay. To correct these anomalies, physicists have developed refocusing techniques such as dynamical decoupling and quantum error correction. Therefore, coherence time plays a pivotal role in the performance of QC systems.
Crosstalk
Crosstalk – a major type of destructive noise – is another factor that affects the performance of QC systems because it hinders the interaction between the qubit and its environment. Crosstalk is a wide range of physical phenomena that varies in QC systems.
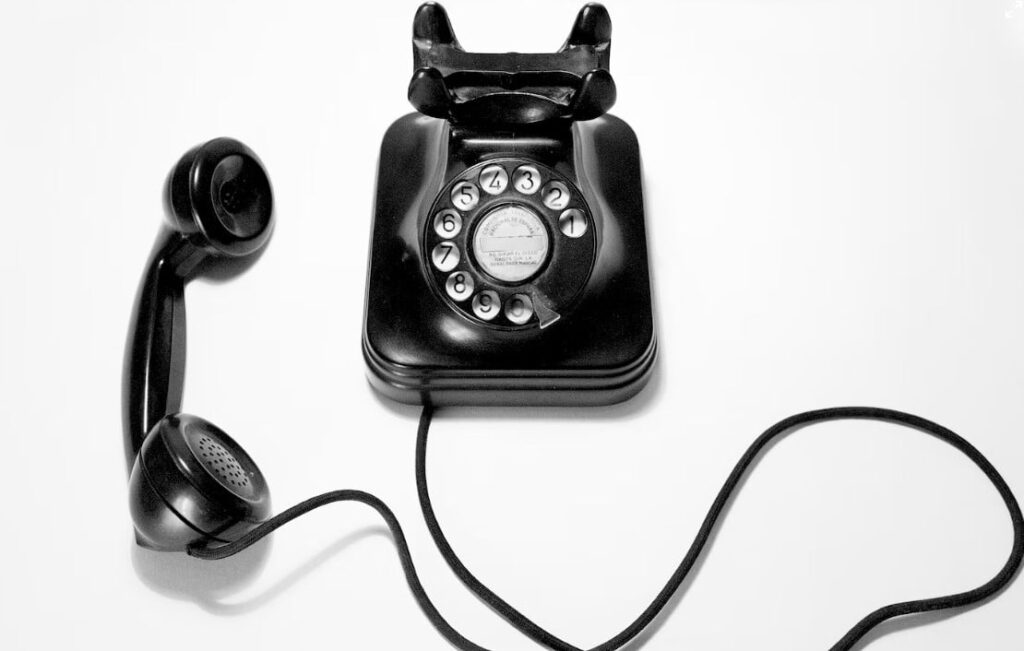
If a QC system has more than one qubit, crosstalk errors are bound to happen. To mitigate crosstalk errors, the errors must be understood, modeled, and detected; that is the means of achieving fault-tolerant computing. Computer engineers build qubits using superconducting circuits. They also need to send electrical or magnetic signals to interconnected chips in order to control them.
This means that QC systems have numerous control lines close to one another in the same chip, which facilitate complex computations. To be useful, QC systems must control and process the information stored in them. But when the number of qubits increases, noise, and crosstalk increase the error rate rapidly; those also inhibit their overall performance.
Gate Fidelity
Gate fidelity is the measure of the closeness of two quantum states. Simply put, fidelity is all about getting correct results and minimizing errors. While attaining 100% fidelity is always the goal, achieving it in reality is a tough nut to crack. In most QC systems, fidelity is a continuous spectrum from low levels to high levels.
The idea behind fidelity is taking the two copies of a state, implementing a gate on one copy and applying the ideal gate on the other, and computing the fidelity between the two results. A relatively high-performing QC system must have 99% fidelity or more. According to Andre Morello, the Scientia Professor of Quantum Engineering at the University of South Wales, 99.9% fidelity has been achieved on individual qubit operations.
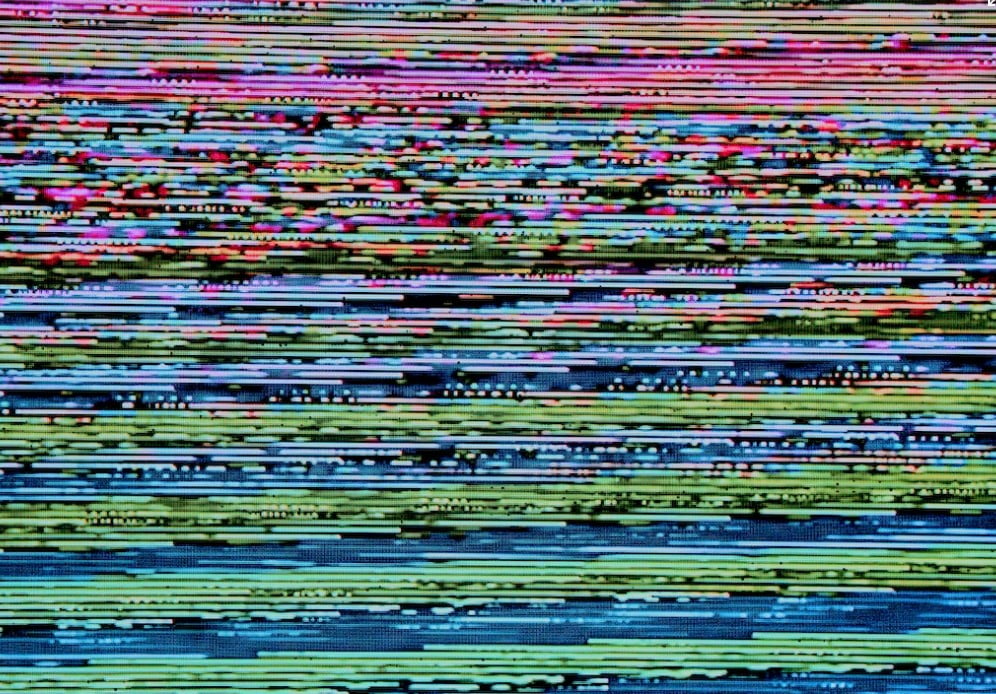
Sadly, the addition of more qubits lowers the system’s fidelity. Although a topnotch-performing QC system doesn’t necessarily have to run at 100% fidelity, anything lower than that will result in errors in QC calculations when multiple operations have taken place. Error-correction protocols are used to mitigate decoherence but these protocols often come at a high cost.
Conclusion
In this article, you have learned that quantum volume is an important parameter that affects the performance of QC systems. In other words, enhancing the performance of such devices doesn’t solely depend on scaling the number of qubits and modality (model).
As regards quantum volume, a lot of factors are taken into consideration, such as coherence time, cross talk, and gate fidelity. Other factors that matter in quantum volume, according to IBM, are spectator error, coupling map, initialization fidelity, gate parallelism, circuit optimization, and calibration errors. Interestingly, we have discussed the most critical factors in this article in the hopes that you now have a better idea of what makes the difference in the overall performance of quantum computers.
To answer the question, increasing qubits does not necessarily enhance the performance of quantum computers because factors like quantum volume and modality also affect their overall performance. Hopefully you know have a basic understanding to the primary question in this article, Does Increasing Qubits Improve Quantum Computing Performance.