Measuring Quantum Computing Performance
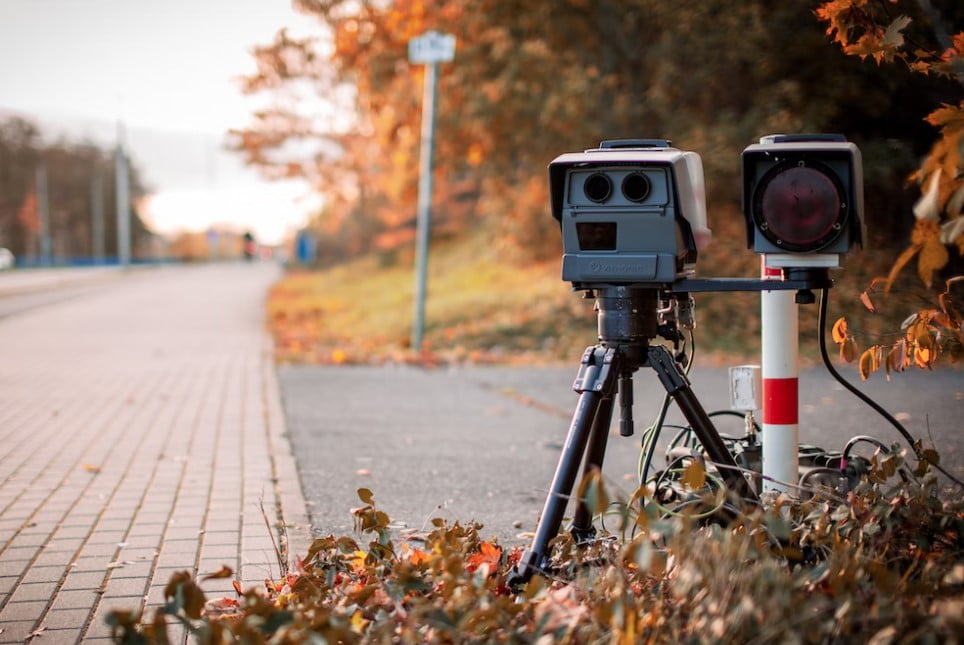
Even though quantum computing (QC) is at the infancy stage, it is fair to say that we already have an idea of what the machines are capable of doing. While the research into QC systems is in top gear, there are various architectures out there and they are progressing at different rates. But then, the capability between each modality varies along with the associated performance.
Often asked “How to measure Quantum Computer Performance” find the answers in this article.
Basically, QC performance is the amount of useful work such machines can do per unit of time. Over the years, authors have published articles and journals, assessing the performance of quantum machines. In most of the publications, the number of qubits was used to measure the performance of QC systems. While this provides some useful information about the next-gen technology, there is more to it. So, you will learn the three major metrics for measuring QC performance in this informative article.
SCALE (QUANTITY)
Scale or quantity is the number of qubits in a QC system because it determines the size of problems they can solve. Sure, this is what those articles taught us. To manufacture better computers, computer engineers must keep increasing the size of QC systems at all times.
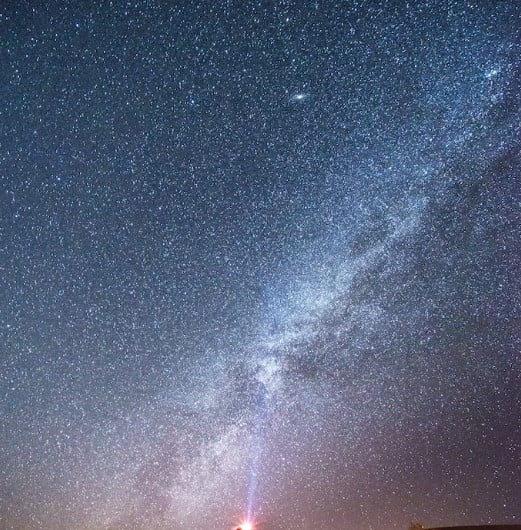
For instance, in 1998, Isaac Chuang of the Los Alamos National Laboratory, MIT and Mark Kubinec of the University of California created a 2-qubit quantum device that could be loaded with data to process data and output a result. Later in 2021, IBM unveiled the world’s most powerful quantum machine (in terms of number of qubits), which has 127 qubits.
Put simply, it took the researchers and developers over two decades to “scale” to 125 qubits. IBM’s next plan is to scale to 433 qubits and research is underway to bring that idea into reality in 2022.
From the foregoing, increasing the quantity of qubits has been a tradition in the industry. While universal gate developers are looking to develop 433 qubits this year, quantum annealing developers, such as D-Wave, have already hit 5000 qubits.
For what it’s worth, this means that scale is not just enough for determining performance of these hi-tech machines because there are different QC architectures in the domain. Guess what, there are other reasons!
As we continue to navigate towards an answer to How to measure Quantum Computer Performance, its always good to remember, we are in early days and its likely over the next 3-5 years more substantive systems will be in place to assist with measuring quantum computing performance and quantum volume.
QUALITY
All the talks about QC systems will amount to nothing if the developers don’t build high-quality machines. Factors that determine the quality of quantum machines are material loss, readout errors, controls and other imperfections.
To measure the quality of these disruptive technologies, quantum volume (QV) is used. The quantum volume determines how faithfully a quantum circuit – basic unit of computation in QC technology – can be implemented in a system. Simply put, QV is the biggest random square circuit that a quantum processor can successfully run. To improve the quality of QC systems, it is also crucial to improve coherence, gate fidelity, measurement fidelity and cross-talk.
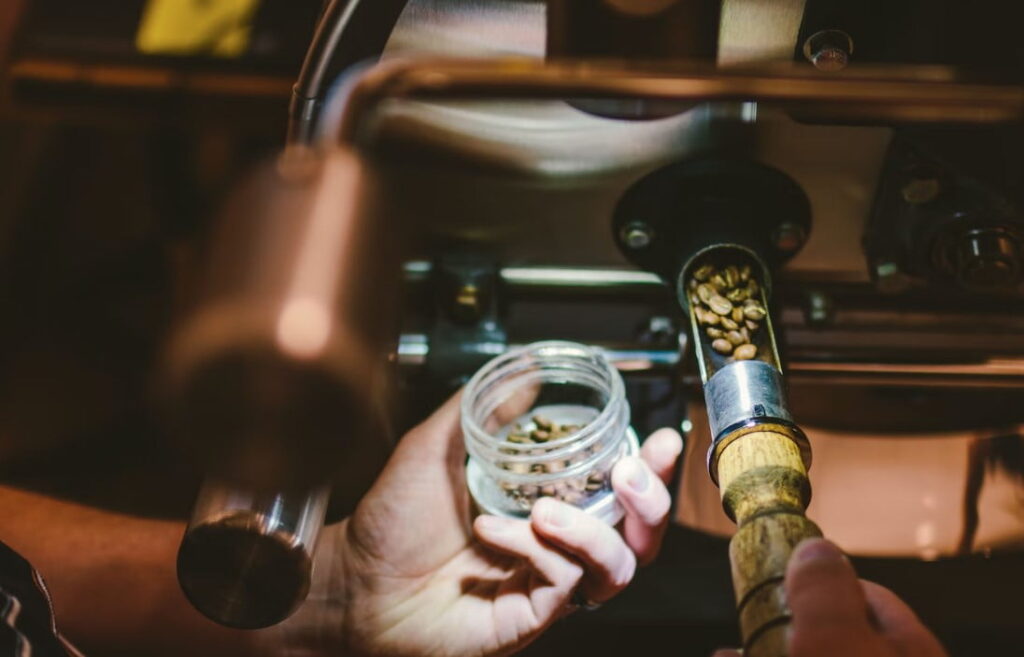
On the other hand, connectivity and gate parallelism largely affect quantum volume. Readout is the method used to verify the authenticity of a secure object.
As QC technology continues to make progress, noise plagues the innovative system. Noise is due to imperfect control signals, interference from the environment and unwanted interactions between qubits. Indeed, noise leads to high error rates.
What this means is that a clean 50-qubit QC machine is likely to outperform a noisy one that boasts 500 qubits. Yes, the performance of QC systems does not only depend on scale or quantity as you have seen the import of quality in the calculus.
The good thing, nonetheless, is that researchers, such as those behind QuantumNAS at MIT, are currently working on various projects to develop a noise-adaptive framework. The idea is to develop parametrized circuits of adjustable quantum gates.
SPEED
Speed is another factor that affects its performance. In fact, if “quantum supremacy” is ever going to become commercially viable, system developers must work on the speed of quantum machines. In simple terms, QC speed is the number of primitive circuits that can be processed per second. Increasing QC speed means revving up the quantum processing unit (QPU).
Moving on, in a nutshell, you can improve QPU speed through the following:
- The gate and readout must be fast so they can operate quickly. The physical architecture somewhat drives this component. Hence, many QC companies deploy superconducting qubits in their systems because they offer fast gate operations and readout. More importantly, the developers can engineer strong couplings between qubits and readout resonators to achieve their goal.
- Building advanced control electronics that allows qubit reset for reuse. To achieve this, IBM, for instance, uses FPGAs (field-programmable gate arrays). FPGA is an integrated circuit designed to allow the user to program it from their end.
- Reducing latencies and increasing the performance of software stack. This is achieved through relevant algorithm generation, pulse orchestration, instrument loading and efficient interaction with the cloud using Qisit Runtime API.
Furthermore, QC speed is measured using CLOPS (Circuit Layer Operations Per Second), which is the measure of how fast a quantum processor can execute circuits. There are lots of hardware-software stack factors that contribute to CLOPS, such as repetition rate of the quantum processor, the runtime compilation, the amount of time it takes to generate the classical control instructions, the speed of the gates, and data transfer rate among all the components.
CONCLUSION
As researchers work round the clock to learn more about QC technology, the world gets a clearer picture of how the technology works. In the past, for instance, many people erroneously believed that only the number of qubits determines the size or complexity of the problem it can solve. As you have learned in this article, such information is misleading because QC performance depends on three factors: scale, quality and speed.
Yes, scale (number of qubit) is an important factor in the equation but not everything. Despite that, it must be established here that scale often improves quality and speed of QC systems because extra qubits can be used to boost the circuit-processing performance of QPUs by multiprogramming them.
Indeed, having an in-depth understanding of what matters in the QC world will help future enterprise users to make informed decisions when the powerful machines finally go into full-scale commercialization.